AD ASTRA: The life of a star
- Alessia Palazzo
- Articles
- 6 minutes (1177 words)
The night sky. So dark, cold and empty. But if you find yourself far away from the city madness and dare to look up, you would realize that it is not empty at all. Tiny, shiny dots begin to appear as your eyes adapt to the darkness. Stars brighten the emptiness of outer space and their light seems eternal. Well, for us, it is, since our lives are immensely shorter than the life of a star. But have you ever wondered what living that long would be like? No? Well, let me take you through the life journey of a star.
Ready? AD ASTRAAAAA
We begin our journey in the womb of stars, which is called a nebula. Nebulae are huge clouds of gas and dust that collapse under their own gravity. Denser regions attract more matter towards the core. This process is known as accretion and self-amplifies until the cloud heats up and becomes a protostar.
In the core of the protostar, matter is compressed and reaches very high temperatures. Elevated density and temperature stimulate nuclear fusion, which produces light. This is called stellar ignition and can be seen as the star’s birth (but instead of a few hours, it lasts for 500,000 years, which must be painful for the mother). However, not all protostars will make it: only a core temperature of 10 million K will allow efficient fusion of H into He, thus ensuring the star’s survival. As Darwin teaches us: life is a struggle and only some can survive. In this case, may the hottest among us win ;)
However, being hot is not the whole story. You also need to be massive: generally speaking, stars below 0.08 solar masses will not make it and they will become brown dwarfs or failed stars. Hey but don’t worry, failed stars also shine bright for a few million years!!
On the other hand, protostars that are sufficiently hot and massive to ensure a steady nuclear fusion will become main sequence stars. These do not collapse under their own gravity because the energy from the core generates an outward pressure that counterbalances gravitational attraction. Despite the name “main sequence”, these stars are not homologated and without personality. Instead, they have different spectral types (based on temperature, mass, size, brightness) which translate into different colors. These are: blue (O), light-blue (B), blue-white (A), white(ish) (F), white-yellow (G), orange-red (K) and red (M).
Fun fact: a nice mnemonic to remember spectral types is: Oh, Be A Fine Girl/Guy and Kiss Me!
We can picture a main sequence star like an “adult star” with its life figured out and continuing to do its job: shining. However, stars also get old and tired and eventually die. The lifespan of a star depends on its mass: the more massive the star, the shorter it lives because it consumes H faster. So, being hot and massive may help you in the beginning to start shining, but it reduces your time as a big bright star.
When the star’s fuel runs out, nuclear fusion ceases and there is no force to counterbalance gravity. The star collapses and the core becomes hotter and hotter, eventually pushing outwards the outer layers. The star has overworked itself and now is the time to retire as a red giant. At this point, the core becomes more unstable and different fates await our red giants based on their mass.
Average stars (up to 1.4 solar masses) continue to eject the outer layers until the core is exposed. These become hot, but small, white dwarfs. They don’t collapse further because fast moving electrons in the core create a counterbalanced pressure to gravity. White dwarfs will eventually cool down and fade away. Sad :(
If the star’s mass exceeds 1.4 solar masses, the electrons cannot generate enough pressure to fight gravitational attraction. If the star is part of a binary system, gravity will pull in closeby materials and gas (H) from the companion star. When enough H has accumulated on the surface, the outer layers explode in a burst of nuclear fusion. This causes the white dwarf to brighten and expel materials. After a few days, the glowing fades away and the cycle starts again. The periodic increase in brightness followed by fading is characteristic of novae and caused by the explosion of their outer layers.
If the star is even more massive, it attracts more matter and eventually the core collapses and explodes completely. This results in a supernova. The collapse of the core of a star releases an unimaginable amount of energy and the light emitted may outshine an entire galaxy. However, in a galaxy, a supernova explosion occurs on average “only” once every 100 years.
After a supernova explosion, there are different scenarios. If the mass of the star is between 1.4 and 3 solar masses, the core will collapse until protons and electrons combine into neutrons. This results in incredibly dense neutron stars. They have strong magnetic fields that accelerate particles into beams of electromagnetic radiation and, if they are spinning, these beams will reach us at regular intervals. This is known as a pulsar.
You all know what comes next. If the mass of the core exceeds 3 solar masses, the star will collapse into everyone’s favorite: a black hole. This is a region of spacetime with infinite density, where gravity is so strong that nothing can escape, not even light. But black holes are not as scary as you might think. They do not swallow everything they encounter like a cosmic vacuum cleaner. If you maintain the proper distance (further from the event horizon), a black hole is not any different from other massive objects.
But wait a second, if black holes absorb all electromagnetic radiation, how can we see them? How do we know about their existence? Black holes are detected because of their powerful gravitational field, which attracts materials from nearby stars and forms an accretion disk. This contains hot gas that emits X-rays or gamma rays which we can detect.
P.S. This is not the actual death of a star because black holes evaporate through Hawking radiation, the theoretical evaporation of a black hole. Without getting too much into incomprehensible physics, basically quantum weirdness leads to the production of pair-particles (a particle + its antiparticle) and one of the two crosses the event horizon. Then, to justify the existence of the companion, the black hole loses energy as Hawking radiation.
So, is this the end of the story? Not entirely, because “what goes around comes around”. The dust and debris left after a nova or supernova explosion contains heavy metals that mix with interstellar dust. These heavier elements are recycled by the next generation of stars or are used to form other celestial objects like planets and asteroids. Some of this stellar waste also created our Earth and organic molecules which eventually became life. In the end, we are all just stardust.
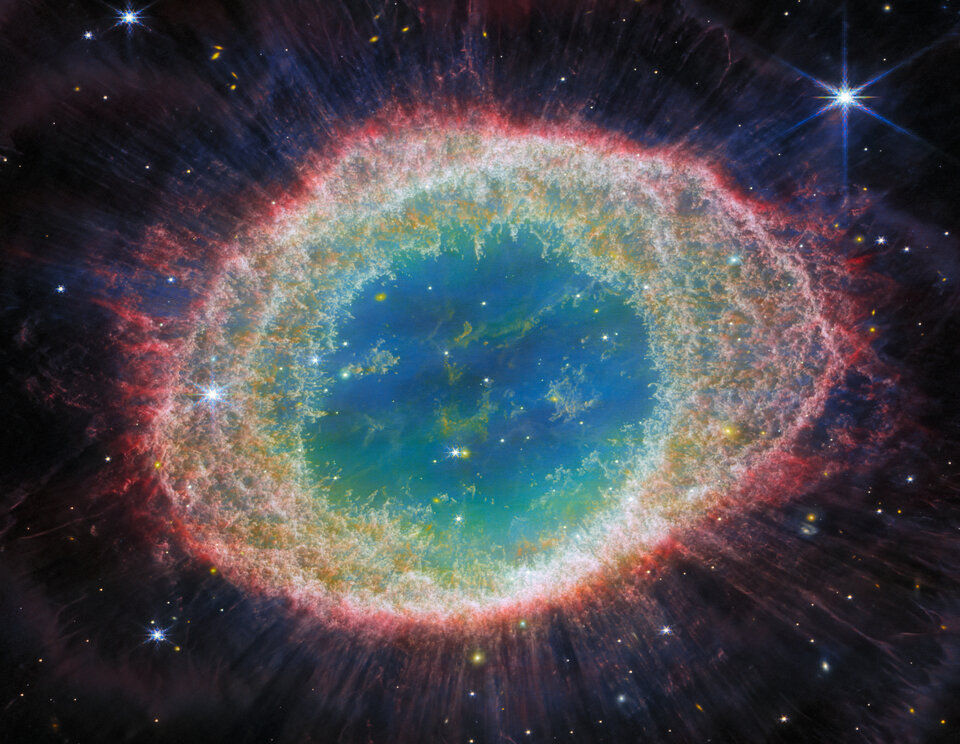
Comments
Log in to read and post comments